Lagunita Theme
Clean hydrogen production
Molecular hydrogen can serve an important role in the clean energy ecosystem, serving as fuel due to its high energy density, a chemical precursor for fertilizer, and long-term energy storage among other applications. If produced using clean, renewable electricity, the carbon footprint of hydrogen fuels can be extremely small. The dominant process to generate hydrogen from clean electricity is known as electrolysis, in which an applied voltage splits pure water into hydrogen and oxygen gases. While this method has great potential, it currently is not yet cost-competitive with hydrogen produced from fossil gas, a process which generates significant emissions. Improving the efficiency, safety, cost, and durability of electrolyzers is a necessary step to scaling up production and usage of clean hydrogen.
Gas crossover in proton-exchange membrane electrolyzers
Operating proton-exchange membrane electrolyzers at high current densities, under high differential pressures at the hydrogen outlet, and using thin membranes can significantly improve the economics of hydrogen production. However, these conditions all increase the permeation of oxygen and hydrogen through the membrane, which create safety, durability, and efficiency concerns. We are using continuum models to study the physics driving gas crossover and to optimize crossover mitigation strategies such as recombination catalyst layers.
Batteries & the lithium-electrolyte interface
Lithium metal anodes are required for high energy density next-generation batteries, with applications in electric vehicles, grid-scale storage, and personal devices. However, during charging, spontaneous growth instabilities known as “dendrites” can lead to short circuiting and electrolyte degradation, causing significant safety and performance concerns. My work focuses on using simulation and theory to develop our understanding and control of the lithium-electrolyte interface towards long-lived, safe lithium metal batteries.
Polymeric interphases for lithium metal batteries
Soft polymeric interphases between the lithium metal anode and the electrolyte have been shown to suppress dendritic growth, overturning the predictions of existing theoretical approaches. We use coarse-grained molecular simulations and develop analytical theory to understand how polymer permittivity, viscoelasticity, and thickness alter the stability and morphology of electrodeposition. Our aim is to develop fundamental principles for rational design of polymer coatings in electrochemical systems.
Molecular simulation of lithium ion solvation
The solvation strength and the structure of the solvation shell have been shown to correlate with the composition of the solid-electrolyte interphase and Coulombic efficiency of lithium deposition. We develop molecular simulations of novel electrolyte solvents to elucidate the effect of electrolyte chemistry on lithium solvation.
Linear stability analysis of lithium metal deposition
We are developing the first linear stability analysis of lithium deposition through a polymer electrolyte to include the coupled effects of ion transport, elastic mechanical response, and Marcus kinetics. This technique determines whether small-scale, randomized disturbances at the lithium-electrolyte interface will grow into large-scale heterogeneous morphologies.
Field theoretical prediction of metal electrodeposition kinetics
We are extending a field theoretical approach to calculate the free energy landscape for the lithium metal electrodeposition reaction at the electrode-electrolyte interface, accounting for the orientational and electronic polarization of a mixed-solvent electrolyte. Free energy curves for the initial and final states along a reaction coordinate allow direct evaluation of the reorganization energy and activation free energy which govern the reaction kinetics.
Funding sources
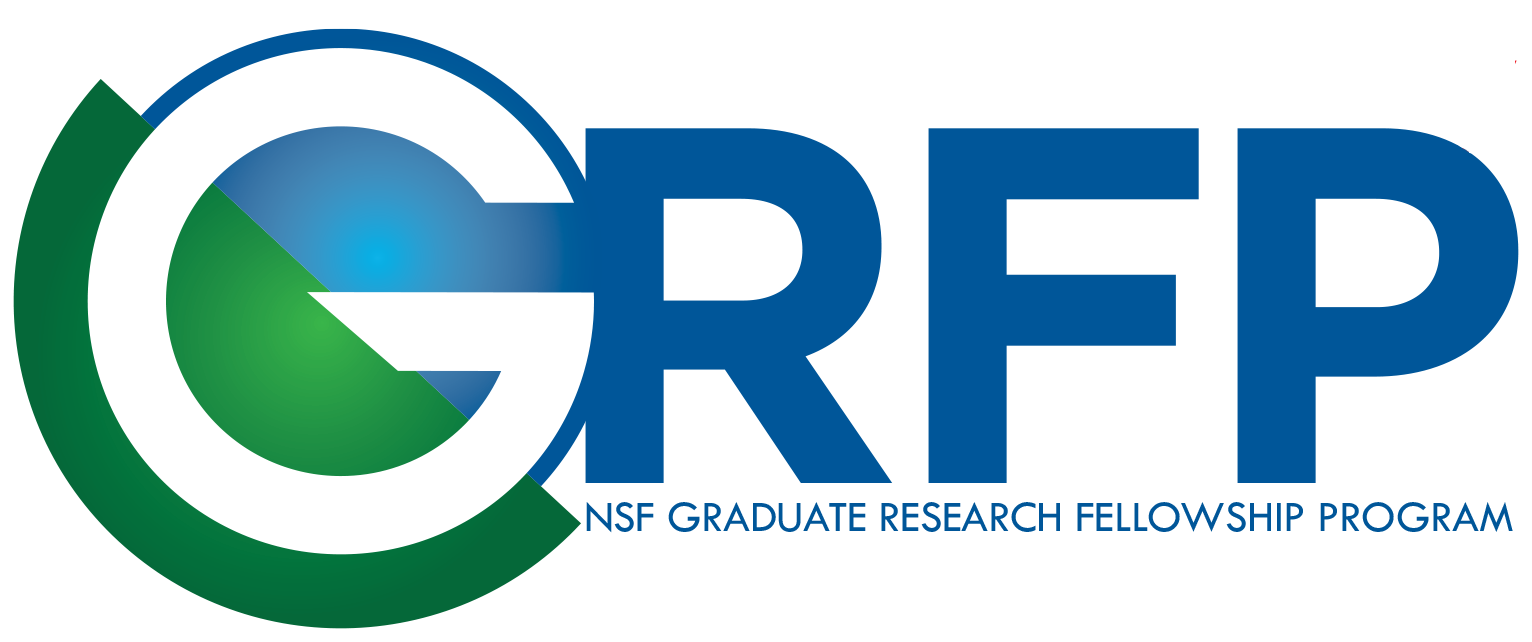
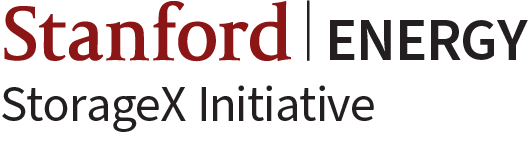
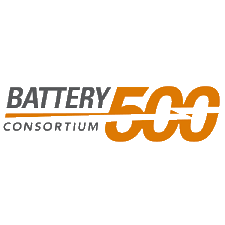
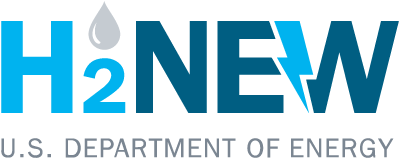
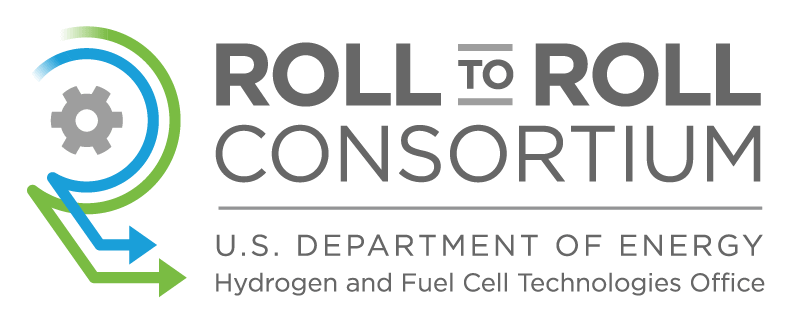
Connect